Download the PDF file
Electronic devices
traditionally produce heat. While there
have been great strides to reduce the power consumption of devices, newer
computers and many other densely packaged electronic items still produce enough
heat to destroy themselves if that heat is not removed. It seems that advancements in low-power,
low-voltage designs are accompanied by greatly increased circuit density, with
much larger processors (more transistors) and more memory. The new electronic devices enable substantial
performance improvements, yet the resulting overall system dissipates as much
power as ever, sometimes even more.
Therefore, a systematic approach to selection and application of adequate
thermal management (usually cooling) is required for all electronic
products. Fortunately there are many
choices.
This paper is written to help
the user make an informed decision on selection of the most appropriate cooling
approach for their system design and application. This guide does not attempt to perform the
engineering required to apply a particular cooling technique, but offers a basic
overview of various choices, and list of considerations to assist in the
selection of cooling approach. And for
evaporative spray cooling, offers numerous nuggets of advice, considerations,
and things to watch out for.
For this paper, the term “evaporative
spray cooling” means a cooling approach that uses some sort of cooling fluid,
sprayed via nozzles or spray caps, onto components to remove heat. The spray mist changes phase (evaporates from
a liquid to a vapor) to carry away heat.
There are many other types of liquid- and air-based cooling approaches
discussed below in comparison and contrast to evaporative spray
cooling.
Cooling
Choices
There are many ways the thermal environment for
electronics can be managed. Many benign
office-like environments may not require any special approach. Simply allowing ambient air to circulate
around the electronics will often suffice to keep the components within
allowable temperature limits, usually 0-70°C for commercial grade
ICs.
Operating in less-friendly
environments, such as a factory floor, or uncontrolled space, forces the design
to have some sort of cooling mechanism other than natural convection. The typical approach is to force convection
by use of fans to move air through the equipment enclosure and around the
components to remove the generated heat.
This works up to a point. Air can
only hold a finite quantity of heat.
Removing more heat with airflow requires either moving more air, or
cooling the air before it enters the system, or both. A large enclosure full of power-hungry
electronics often has several fans roaring away to maintain adequate
airflow. If the available ambient air
reaches a high enough temperature, this approach fails. Increasing circuit density by use of large,
multi-million transistor chips adds yet another dimension to the heat removal
problem.
Designers have long recognized
that changing the heat-carrying medium from air to a liquid holds the potential
for vast improvements (as much as 10X or more) in heat removing capacity. The biggest problem with liquids is that it
adds complexity to the overall system design.
Using air is easy by comparison.
After all, the system is already immersed in it, and moving it around can
be done by using fans and blowers.
Leakage is no big deal. With a
liquid, moving it around not only requires a pump, but plumbing as well. Leaks are not only intolerable, they could
often lead to system failure or damage to nearby items that are not tolerant of
the cooling fluid.
To sum up all the cooling options
available, Figure 1 offers a cooling “family tree” for
consideration.
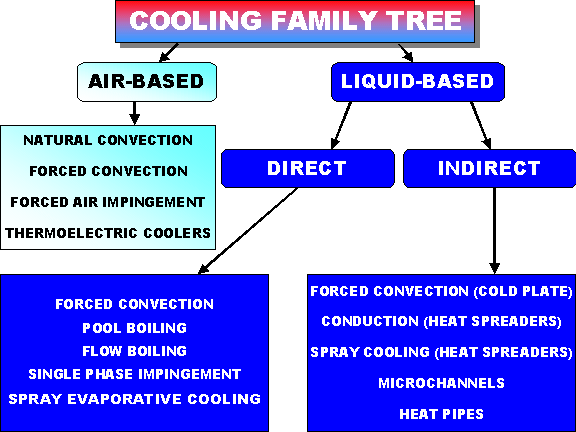
Figure 1. Cooling "Family
tree"
The terms “Direct” and
“Indirect” used above means that the cooling fluid comes into contact with the
components to be cooled (Direct), or contacts another surface that in turn
conducts heat out of the components (Indirect).
AIR vs.
LIQUID
Cost – Air-based cooling is
typically much less expensive than liquid-based. More enclosures are designed around
air-cooling, and fans are typically not very expensive. Liquid cooling enclosures usually cost
significantly more, and the requisite pumps, atomizers, nozzles, and plumbing
add costs and complexity to the overall design.
Evaporative spray cooling, or other novel cooling approach, may enable
use of lower-cost COTS circuit boards in an otherwise harsh environment, which
may offset some of the added integration cost.
Reliability – A fan failure on an air-cooled enclosure
can often be tolerated, especially if some redundancy is designed in. The higher operating temperatures of dense,
heat-generating components in air-cooled designs may lower overall system
reliability. Liquid cooled system pumps
tend to be about as reliable as fans, however a pump failure may be a more
catastrophic event if redundancy or adequate controls and “fail-safes” are not
designed in. Liquid cooling of dense,
heat-generating components tends to lower operating temperatures on the hottest
parts, which may boost overall system reliability. In some liquid-cooled systems the temperature
is about the same throughout (an isothermal design), which has led to much
debate about whether this helps or hurts system reliability (some low-power
parts actually run hotter than in a comparable air-cooled system).
Maintenance – Maintenance of air-cooled designs is very
straightforward and familiar to most technicians. There are no fluids to drain, plumbing to
unhook, or seals to check. The liquid
cooling designs add extra steps to system maintenance procedures. There is debate on whether the improved
reliability and environmental isolation of liquid cooled designs offsets the
added maintenance needs, since the fluid helps keep internal components cleaner
and cooler (leading to less corrosion, contamination, and operating
failures). Most engineers have concluded
that organizational or even interim depot-level maintenance is not suitable for
evaporative spray cooling. Most suggest
using only a well-equipped depot operation.
Performance – Air-cooled
systems have a much lower total heat capacity than liquid-cooled systems of the
same size. While additional space must
be devoted to a heat exchanger in a liquid-cooled design, this exchanger may be
located separately from the functional system, allowing for some
flexibility. This may actually be
considered a benefit of liquid-cooled designs, since the collected heat is
transferable to a remote location. In
air-cooled designs, the system produces local area heating, which may put
additional strain on area air conditioning.
This is often not calculated in considering the overall space needs of
air-cooled designs, as the air conditioning system of the room the system is in
may need to be larger. Liquid-cooled
systems can be environmentally isolated easier than air-cooled designs, which
may permit their use in severe operating conditions without much change. Air-cooled designs, since they draw in large
quantities of external air, cannot be used in hot, dirty, or water-soaked areas
without careful adaptation to deal with the poor quality intake air. A liquid-cooled system could potentially be
used in a complete vacuum, such as outer space.
Table 1. Comparison of various
Air- and Liquid-based Cooling Approaches
Decision
Tree
§
If air-based cooling will meet your
requirements, it is probably the best way to go. It is by far the less expensive and easiest
to implement. The simplest is natural
convection. Next is use of fans to force
convection.
§
If air cannot meet all your requirements, then a liquid-based
approach may work, but be prepared for additional costs, some additional design
considerations and limited system layout and construction
options.
§ The
most complex liquid cooling options - evaporative spray and microchannel – are
the most costly and difficult, yet provide the highest cooling
capacity.
§ A
combination of approaches for a larger system may be the optimum solution,
applying the complex and costly techniques only where needed, and using
traditional air cooling for the balance of the
system.
Various Liquid Cooling
Issues
There are numerous
choices to make in selection of a liquid-cooling approach. Below, some of the many considerations are
discussed, along with some suggestions.
Direct or Indirect Fluid
Contact?
Also called an “open”
(direct fluid contact with electrical components) or “closed” (fluid kept
separate from components) system. With
direct liquid cooling, the cooling fluid contacts the components to be
cooled. With indirect, the cooling fluid
does not contact the components, but conducts heat away from a cold plate or
heat spreader the components are mounted on.
As one can guess, a direct contact fluid must be a dielectric fluid. Indirect fluids do not have to be
non-conductive, and can even include water.
Some issues to consider
–
F
Direct contact possibly eliminates the need for a heat sink or
cold plate. Many commercial boards
designed for air convection cooling can be adapted to direct cooling by removal
of the heatsinks. With indirect, the
air-based heatsinks may need to be changed to ones compatible with the indirect
fluid cooling approach – a likely expensive and difficult step. A custom circuit board design with an
integral heat sink or cold plate may be required in some approaches, such as
microchannel.
F
Direct contact means fluid compatibility issues. Some cooling fluids may react with (dissolve
or weaken) the component packaging materials, such as plastic, epoxies, or
metals. Indirect cooling usually keeps
the fluid separated from sensitive materials.
Keeping the fluid contained also means potentially less filtering and
contamination problems.
F
If an open system is not intended
to run for extended periods of time there are additional things to consider. It
may be less expensive and lighter to have a pressurized reservoir to deliver the
fluid to the electronics to be cooled versus a pump and reservoir. The fluid may
not even need to be collected if the electronics being cooled have a limited
lifetime, this could potentially eliminate a heat exchanger and other
components, resulting in space and weight gains as well as a reduction in
cost.
Evaporative Spraying
(change-of-phase), or Single-phase Liquid?
Evaporative spray cooling uses
nozzles to direct a fine mist of coolant onto selected components. The heat from the components is removed by
the evaporation of the coolant mist into a vapor. The vapor is then condensed and reused or
simply removed from the immediate area.
Single phase cooling
involves spraying or pumping a liquid coolant directly over hot components. The idea is to provide a fast-moving stream
of cooled inert liquid directly on a high heat-dissipating device to literally
flush away the heat. The coolant is then
collected and re-circulated. Some
spraying approaches are also called jet impingement, since the coolant spray
resembles a jet blast in miniature.
Some issues to consider –
F
The use of evaporation (change-of-phase from a liquid to a gas) to
collect heat from power-dissipating components yields significant increases over
the use of single-phase fluid conduction.
This approach is discussed in detail in the section below on evaporative
spray cooling.
F
With jet impingement, there are problems in properly positioning the jet
spray and the erosion of delicate components by the high-speed fluid
jet.
F
With either approach there is extra equipment required for pumping,
collecting, and filtering all the coolant, unless the coolant can be discarded
by some means outside of the system.
Pool Boiling and Flow
Boiling
These techniques involve total immersion of the electronics in a
specially designed dielectric fluid. The
fluid is designed such that the boiling point is sufficiently low to have the
heat transferred away from the hot components when the fluid boils near
them. These techniques are rarely used
for computers, and find most applications in power systems.
Conduction Cooling (Conduction to
a “Cold” Plate)
Chilled water cabinets have been in use for years in the
Navy, using conduction cooled electronic boards that move the dissipated heat
out via metal frames, cold plates, and heat pipes, rather than by moving air
directly over the boards. The ship
provides a chilled water source that is plumbed through large electronics
enclosures, and returned to the chiller for heat removal. High power dissipation devices such as TWTs
in radar systems and rectifiers in large power microwave transmitters are
similarly cooled. At the microcircuit
level, the heat dissipating components are mounted on a frame designed as an
integral part of the printed circuit board.
This metal frame, usually an aluminum or copper alloy, conducts the heat
to side rails that fit tightly into the cabinet slot. The enclosure is built with cold plates
constructed with channels for circulation of the chilled water within. There is little need for air circulation
inside the enclosure as the heat conducts through the metal parts and into the
chilled water.
Alternatives to
water include PAO (Poly-alphaolefin), Ethelyne Glycol & Water mix (EGW), and
other “engineered” fluids, including 3M's Fluorinert. Designs for aircraft and other applications,
where chilled water is not available out of a tap, often use a closed system
filled with one of these alternative coolants.
Some issues to consider
–
F
The move to COTS has pretty much squelched designing with this approach
for some systems because many COTS boards today are designed to use air-based
cooling. “Rugged” COTS products offer
extended temperature range operation using a conduction cooling approach, but
many still depend on air convection cooling.
There are limited COTS boards that make use of a frame compatible with
conduction cooling, and these tend to be much more expensive than their
air-cooled cousins.
F
Chilled coolant promotes water condensation in humid environments, so
drips and puddles of condensed water must be expected. Most chilled cabinets use a drip tray to
route the condensation to a collection point.
One fix for this is to keep the coolant at a reasonably warm temperature,
although that may be unsuitable if the electronics generate too much
heat.
Evaporative Spray Cooling – Special
Design Considerations
Evaporative spray cooling has found its way into several applications to
cool high-power, or heat-dense components such as power diodes and transistors,
large processor multi-chip arrays, and tightly packed circuit cards. While spray cooling can be applied to
numerous other designs, the increased design and equipment cost, added size and
weight, and loss of maintenance flexibility usually tilts cooling designs toward
more traditional air-based cooling where possible. For these reasons, spray cooling is typically
considered only if traditional air simply cannot meet requirements.
Designing
an effective evaporative spray cooled system must include all aspects of the
total system, not just the spraying equipment.
Assembled below are sections that address these concerns, and the various
items to consider are discussed to illustrate the potential problems that must
be overcome before a successful evaporative spray cooling design can be
achieved.
A Hermetic or Non-Hermetic System?
A hermetic system is completely sealed against infiltration
from outside contaminants, such as water, dirt, or gas. A non-hermetic system may allow some
infiltration, and the system is usually tolerant of the contaminants, or has
some means to deal with them. Any type
of fluid cooled design can be made hermetic, a characteristic with which
air-based cooling has difficulty.
Some
issues to consider –
Hermetic System
|
Non-Hermetic System
|
Costs more
|
Costs less
|
Maintenance is hampered by additional seals and
control of access
|
Maintenance access is simpler
|
Needed for control of gas or moisture
infusion
|
Cooling fluid prone to buildup of contaminants
over time
|
Controlled pressure inside (usually positive vs
external)
|
Lower cooling capability than hermetic
|
Heat exchanger design easier
|
Heat exchanger design more difficult
|
A Gravity-Dependent or -Independent
System?
With a gravity-dependent system the orientation is
fixed, and gravity helps draw fluid down into a reservoir for collection and
redistribution. A gravity- (or
orientation-) independent design can be positioned in any orientation, and fluid
spray and collection become bigger problems.
Some issues to
consider –
Gravity-Dependent
|
Gravity-Independent
|
Fluid
collection aided by gravity, system orientation must be somewhat fixed.
|
Space
and airborne applications, no gravity needed for fluid control.
|
Simpler
fluid collection and spray nozzle design.
|
More
complex fluid collection and spray nozzle designs.
|
Pressure of vaporization assists in the removal of fluid from the area
being cooled.
|
Useful
when the design does not allow for pressure of vaporization to aid in the
removal of coolant from the area being cooled.
|
Pressurized (Gas saturated) or
Non-Pressurized System?
Pressurizing the system has
a few advantages, but can also lead to a bit more cost and complexity. By maintaining a positive pressure inside the
system enclosure, external contaminants tend to be kept out. Also, a pressurized system can be used to
more accurately control the component operating temperature.
One way to control the system
pressure is though the use of nitrogen (or air if corrosion is not an issue).
Part of the nitrogen gas saturates into the fluid while the rest remains in a
gas form in the void space of the closed system. The additional pressure that
the nitrogen gas exerts on the fluid causes a state of “gas subcooling.” The
pressure of the nitrogen can be adjusted, there-by adjusting the boiling point
of the working fluid. The are also other effects that occur as the nitrogen
levels in the fluid decrease as the fluid is heated when it comes in contact
with the hot surface. The over-all effect is that the fluid can remove higher
heat fluxes but the surface temperature also increases for a given heat
flux.
Some issues to
consider –
Pressurized System
|
Non-Pressurized System
|
Needed
to carefully control system operating temperatures.
|
Use
when precise system operating temperature control is not required.
|
Use
when positive internal pressures or positive head is required on pumping
system.
|
Use if
positive pump head is not needed.
|
Positive pressure works to keep air and gas infiltration down.
|
Use
when evaporation temperature effects on operating pressure of no concern.
|
F There are UL
requirements for a pressurized system for safety and operation purposes for
commercial applications. The military
typically adheres to these same requirements.
An overpressure safety release valve is typically required on pressurized
systems.
Pump and Nozzle
Selection
Selecting the
right pumps and nozzles to use involves a large tradeoff between mechanical,
thermal, and electrical power properties.
The pumps must supply adequate pressure to the nozzles to achieve the
desired coolant flow and spray patterns.
Controlling the coolant flow to the nozzles, meeting sufficient thermal
efficiency, while also keeping electrical power use and noise to a minimum is a
very involved problem. There are
numerous pump designs to choose from, three of which are compared below.
The quantity of nozzles needed, the capacity of the
pumps to supply adequate pressure and fluid flow, and the electronics layout
that is to be cooled all affect the combined nozzle/pump design. The electronic packaging and layout of the
system design might preclude or limit certain spraying approaches. For example, a circuit board with only a
large BGA style package to be cooled may only require a single spray nozzle,
while a multi-chip module may require a custom spray plate consisting of several
nozzles. The spray pattern needed for
coverage of the area to be cooled must also be factored into the nozzle
design. Spray cooling packaged single
chips such as a processor must be approached differently than customized
multi-chip modules.
Spray cooling is less effective at reducing
operating junction temperatures in a packaged chip than if the spray can be
directed on the bare die. The best
spray cooling advantages are realized when the spray cooling design can be
integrated in the total chip and system packaging scheme. Adding spray cooling to an existing enclosure
design cannot possibly achieve similar efficiencies.
Some issues to consider
–
F
Pumps
and nozzles, just like many electronic devices, are often single source
items. In fact, numerous designs would
be considered custom or proprietary, and substitution with a different
supplier’s pump or nozzle may be out of the question.
F
Overall fluid control is heavily dependent on proper pump and nozzle
combinations. The pump may cavitate if
there is improper fluid pressure, leading to gas in the fluid lines and a drop
in flow.
F Pump Selection and
Requirements: What parameters to control?
-
Flow rate
-
Pressure differential
-
Temperature
-
Heat exchanger performance
-
Current draw
-
Pump
speed
-
Is there positive head on the
pump?
-
Seals and fitting compatibility
-
Pump
control features
Pump Types
|
Advantages
|
Disadvantages
|
Gerorotor Pump
|
Size,
Delivery Pressure, Control
|
Thermal
Load, Cost
|
Gear Pump
|
Delivery
Pressure, No Thermal load, Control, Reliability, Fluid
compatibility
|
Size,
Cost, Contamination
|
Centrifugal Pump
|
Cost,
No Thermal
load
|
Reliability, Sealing,
Control
|
F
Larger
system designs employing spray cooling across more than one chassis may want to
consider a “common pump network”, where a single set of pumps supply cooling
fluid to each chassis. This approach may
reduce system complexity, but fluid metering and regulation must be employed to
keep all chassis operating within temperature.
F
Spray Plate Design
Considerations
-
What
are the Heat Flux requirements?
-
What is the operating heat flux range?
-
What is the maximum chip heat flux?
-
Is this a gravity assisted fluid removal spray plate?
-
If
more than one chip, what are the interacting dependencies?
-
Is a single nozzle or multiple nozzles required?
-
What is the distance between the nozzle and the cooling
surface?
-
Is the fluid that is being atomized saturated with a
gas?
-
Is flow rate a concern or consideration?
-
Equal flow to nozzles in a multiple nozzle configuration
-
Is full field coverage required?
This is the pattern of the spray. either shaped a certain way (such as a
hollow cone shape) or a solid coverage spray pattern.
Filtering
Requirements
There are several conditions that require filtering the
coolant. Most relate to keeping the
coolant’s operating characteristics within specifications, preventing pump
clogging and wear, and preventing the buildup of unwanted chemicals or
contaminants in the coolant. Most spray
cooling designs make use of at least a particulate filter to remove abrasive,
clog-forming particles. The need for
filters adds to maintenance concerns, but going without them may lead to severe
performance and safety problems.
Gas Filtering
Requirements
-
Prevent the buildup of non-condensable gasses in the system
-
Prevent corrosion
-
Infusion of air and gases is not permissible
-
Material compatibility issues
Moisture Filtering
-
Prevent the buildup of non-condensable
-
Prevention of moisture associated corrosion
-
Prevent heat transfer performance reducing effects from occurring
Organic
Filtering
Particulate Filtering
-
Prevent the fouling of the spraying devices (atomizers and such)
-
Reduce wear of pump
-
Prevent the plugging of other filter types
-
Prevent reduction of heat exchanger performance
Decomposition
Filtering
-
Prevent the buildup of hazardous byproducts,
such as Perfluoroisobutylene (PFIB) from Fluorinert, in the system. (Safety regulation – PFIB can be very harmful
if inhaled or otherwise contacted)
-
Remove hydrofluoric acid to prevent corrosion.
-
Prevent performance reducing effects from occurring from due to
fouling
Fluid Selection Factors
There are many factors to consider when selecting
the proper cooling fluid. Most
spray-cooled systems use a version of Fluorinert by 3M, but there are alternatives,
such as HFE (Novec).
The selection of the cooling fluid should consider the
following topics:
-
Safety - Obviously the fluid must
have the desired properties of boiling point, specific gravity and the like to
be useable, but some accommodation of undesirable events must also be
considered. If a system operates long
enough, a component inside is likely to fail.
Sometimes this failure may be an unspectacular dead chip, but others may
literally “go out in a blaze of glory”.
The fluid must not support a fire (some coolants are actually fire
suppressants), and should not decompose such that it poses a health hazard to
the technician who has to open the enclosure and fix the problem.
-
Material compatibility – Much work has been
done in this area, but obviously no one has tested every material and device for
compatibility with every cooling fluid.
3M has offered to “certify” any design for material compatibility
with the fluid, but this can be an added expense. Individual testing or verification of
material compatibility of selected components in the system design with the
selected cooling fluid should be done.
Other factors to consider: Virgin fluid to material compatibility; Contaminated
fluid to material compatibility issues; Material absorption characteristics;
Material long term property changes; Service requirements; Contamination and
filtering issues Filtering requirements
-
Operational parameters – Most cooling fluids come with some technical
parameters that the fluid has been tested to.
The boiling point, specific gravity, density, and so forth, all can be
selected to optimize performance.
Remember that the fluid freezing point may be high enough to require
system pre-heaters in order to start the system during cold
temperatures.
-
Operating life – A fluid that is expected to last for a long period
of time will likely need maintenance to retain operating parameters.
-
Regulations and governing laws – Some applications do not permit some
of the chemicals used in the cooling fluids.
Better be sure before trying to use them in the application.
-
Environmental release issues – Disposal of the fluid is also
regulated in some cases. Unless you are
using pure water, it is likely that disposal of used fluid will require certain
procedures and special handling.
-
Design application – Engineered fluids for specific design applications
may not be suitable for use in other applications.
-
Heat Transfer requirements
-
Surface temperature requirements
-
Heat Flux requirements
-
Evaporation temperature
-
Filtering requirements – See the “Filtering Requirements” section
above
-
Reliability
-
Cost
-
Availability
Heat Exchanger Design
The heat exchanger can be the external walls
of the system enclosure itself, an internal unit, or an entirely separate
enclosure. Fluid management between the
cooled system and the heat exchanger must be carefully engineered to make sure
the fluid transfer doesn’t generate too much pressure or temperature loss. In any case, the heat exchanger must
effectively remove the collected heat from the system and deposit it in the
desired medium, air or water or something else entirely.
Some issues
to consider –
-
Material selection should be based on fluid selection and performance
requirements
-
Non-condensable in the system determines heat exchanger design
-
Water cooled or air used as heat transfer medium
-
Fluid management in heat exchanger
-
Fluid management in system plumbing
-
Material compatibility issues
-
Serviceability
Summary
If you have
read this far, you can see that there are many options for cooling electronic
equipment, and many issues to consider under each. If evaporative spray cooling appears to be
the most suitable option, hopefully this paper sheds a little light on the
subject and helps to bring up all the concerns that you should answer before
committing to the approach.
POC:
NSWC Crane
Division
Ph. 812-854-6492